Chethana Janith, Jadetimes Staff
C. Janith is a Jadetimes news reporter covering science and geopolitics.
It requires 1 billion times less energy than our current systems.
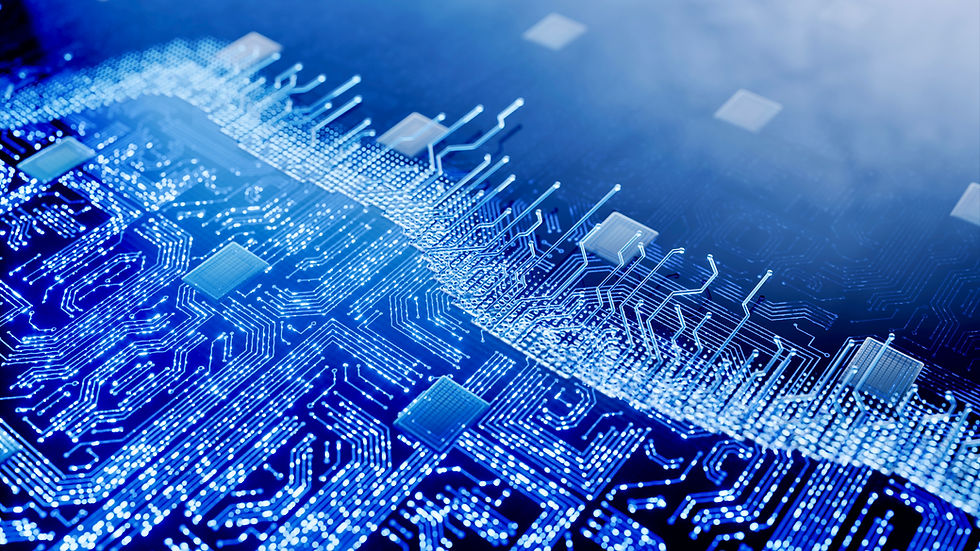
Scientists are searching for a material that could one day bring universal energy - that is, random-access memory and long-term solid-state storage - into one device.
Leading candidates for this material typically requires a process known as “melt quenching” that requires too much energy to be commercially useful.
Now, scientists have potentially discovered a new material: indium selenide, that skips this process and could require up to 1 billion times less energy for storage.
Check out the specs of any laptop at your local Best Buy, and you’ll inevitably come across mention of two types of memory information. The first is random access memory, or RAM, which temporarily stores data for quick access. Basically, the more RAM you have, the more apps can run without incident. The second is a drive - usually a solid-state drive or hard drive, which provides long-term storage even when the device is turned off.
It’s long been the goal of scientists to develop some type of universal memory that can tackle both jobs at once, but the energy required for one of its leading candidates - known as phase-change memory (PCM), has long been too extensive to be practical. That could, however, be about to change.
PCM relies on a process known as amorphization - the arranging of the atoms of a crystalline structure into a new, random structure. Usually, PCM (as its name suggests) needs energy to transform between its crystalline structure and amorphous structure. This requires a technique known as “melt quenching,” which rapidly heats and cools PCM materials. But, of course, a rapidly changing temperature usually requires too much energy to be commercially viable.
Now, scientists at the University of Pennsylvania have developed a new method of amorphization, which uses wires made of indium selenide, or In2Se3. This new technique bypasses that rapid temperature-changing step, and as a result, creates a semiconductor that could require up to one billion times less energy than others of its kind. As the researchers said in a press statement, this is a huge step forward since energy requirements are the main reason phase-change memory devices haven’t reached the mainstream. The results of their study were published in November in the journal Nature.
As is true of many great breakthroughs, the data-storage utility of this inorganic compound was discovered by accident. In 2021, while working in a lab at the University of Pennsylvania and running current through the compound, Gaurav Modi - a doctoral student at the University of Pennsylvania and co-lead author of the paper - noticed that the wire he was working with suddenly stopped conducting electricity. Unexpectedly, the wire had amorphized.
“This was extremely unusual,” Modi said in a press statement. “I actually thought I might have damaged the wires. Normally, you would need electrical pulses to induce any kind of amorphization, and here a continuous current had disrupted the crystalline structure, which shouldn’t have happened.”
Exactly what was occurring at the atomic level in these wires was a mystery that took three years to unravel. It turns out that a few electrical and physical properties embedded within indium selenide powered this strange amorphization process.
“We had to look very, very carefully to understand this process,” Pavan Nukala, an assistant professor at the Indian Institute of Science, said in a press statement. “We learned that multiple properties of In2Se3 - the 2D aspect, the ferroelectricity and the piezoelectricity - all come together to design this ultralow energy pathway for amorphization through shocks.”
The researchers describe this interplay as an electric “earthquake” followed by an acoustic “avalanche.” The material’s piezoelectric properties nudge portions of these layers into unstable positions. At a critical point, these deformations spread rapidly and collide, creating sound waves that resonate throughout the material - not unlike seismic waves after an earthquake. Then, the avalanche hits, as the sound wave (technically called an “acoustic jerk”) drives more deformation and eventually links pockets of amorphization into one mass.
“This opens up a new field on the structural transformations that can happen in a material when all these properties come together,” Ritesh Agarwal, senior author of the study from the University of Pennsylvania, said in a press statement. “The potential of these findings for designing low-power memory devices are tremendous.”
Comments